Assessment of myocardial function and cardiac performance using left ventricular global longitudinal strain in athletes after COVID-19: a follow-up study
- 1Division of Sports and Rehabilitation Medicine, University Ulm Hospital, Ulm, Germany
- 2Clinical & Biological Psychology, Institute of Psychology and Education, Ulm University, Ulm, Germany
Background: It has not yet been conclusively determined whether reduced left ventricular global longitudinal strain (LV GLS) after COVID-19 contributes to a reduction in exercise capacity. Our own studies showed a possible mild myocardial involvement in the form of reduced LV GLS in athletes after COVID-19 compared with healthy athletes. The aims of this prospective follow-up study were to investigate the development of LV GLS over a 3-month period in athletes after COVID-19 and the possible relationship between LV GLS and physical performance.
Methods: LV GLS was determined in four-, two-, and three-chamber views and assessed offline by a blinded investigator in 96 recreational athletes (mean age 33.15 ± 12.40 years, 53 male, peak VO2 38.82 ± 11.14 ml/min/kg) at a median of two (t0) and five months (t1) after COVID-19. Cardiopulmonary exercise testing (CPET) was performed on a bicycle ergometer on both examination dates.
Results: LV GLS improved significantly between t0 and t1 (t0 −18.82 ± 2.02 vs. t1 −19.46 ± 2.05, p < 0.001). Echocardiographic and spiroergometric parameters were within the normal clinical reference range. Maximum power increased significantly from t0 to t1 (t0 283.17 ± 83.20 vs. t1 286.24 ± 85.22 Watt, p = 0.009) and there was a trend toward increased peak oxygen uptake (t0 36.82 ± 11.14 vs. t1 38.68 ± 10.26 ml/min/kg, p = 0.069). We found no correlation between LV GLS and performance parameters, except for the respiratory exchange ratio (RER) [ρ −0.316, (−0.501; −0.102), p < 0.050].
Conclusions: Significant improvement in LV GLS approximately five months after COVID-19 may be due to mild myocardial involvement during or shortly after COVID-19, which seems to recover. There was no correlation between LV GLS and performance parameters, except for an inverse correlation of LV GLS and RER, suggesting insufficient exercise intolerance at lower GLS values. Further studies on the development of GLS in athletes or in the general population with moderate and severe disease courses would be informative as well as the comparison of pre-COVID-19 with post-COVID-19 echocardiography to evaluate the effects of COVID-19 on cardiac function.
1. Introduction
Coronavirus Disease 2019 (COVID-19) is a systemic viral infection caused by Severe Acute Respiratory Syndrome-Coronavirus-2 (SARS-CoV-2) that primarily affects the respiratory system but can also cause myocardial damage (1–3). Studies in elite athletes have shown that the infection is often mild (46%–82%) or asymptomatic (16%–58%) (4–7), with myocarditis occurring only in rare cases (1%–3%) (8–9). Most athletes can return to competitive and amateur sports after a training break adapted to current symptoms (10–12). However, having passed through COVID-19 does not necessarily imply a complete recovery to former health or performance status and a return-to-sport examination with echocardiography should be performed especially in case of cardiac symptoms during and/or after infection (11).
Analysis of myocardial deformation by speckle-tracking echocardiography (STE) can provide additional information to the standard echocardiography examination (13). The global longitudinal strain of the left ventricle (LV GLS) is more sensitive than left ventricular ejection fraction (LV EF) alone in detecting subclinical LV dysfunction (14) and is a prognostic parameter for long-term risk of cardiovascular morbidity and mortality (15). Individual studies demonstrated reduced LV GLS with preserved ejection fraction (pEF) in the setting of acute SARS-CoV-2 infection in hospitalized patients regardless of infection severity (16–19) and in patients recovered from COVID-19 (20–23). No changes in LV GLS have been observed in athletes compared with healthy athletes 22 days (24) and 19 days (25) after COVID-19. Conversely, we demonstrated reduced LV GLS and diastolic function in a cohort of athletes at a median of two months after COVID-19 compared with non-infected healthy athletes (26).
Longitudinally, hospitalized patients showed no significant improvement in LV GLS at two months (27) or three months after acute infection (28), and 25% of patients still had abnormal LV GLS three months after acute infection (28). However, in the follow-up study by Karagodin et al., improvements in LV GLS were noted in patients with impaired baseline function (29). Further long-term observations in athletes are scarce.
The two main aims of this prospective follow-up study were, first, to investigate the development of LV GLS in athletes without history of LV dysfunction at an average of two months after SARS-CoV-2 infection to a follow-up of three months, and, second, to find if there may be a relationship between myocardial changes detected by LV GLS determination and physical performance.
2. Methods
2.1. Study population
Ninety-six recreational athletes presenting to the Ulm Clinic for Sports and Rehabilitation Medicine after COVID-19 were included in this prospective, single-center, longitudinal cohort study after being informed of the study procedures and providing written informed consent. The results presented in this study are from baseline clinical assessment between June 2020 and July 2022, a median of two months (IQR: one to five months) after COVID-19, and follow-up three months later. Study participants were included consecutively. They engaged in endurance sports, strength sports, team sports, or technical sports with a training volume of at least three times per week, corresponding to more than 20 metabolic equivalents of task (MET) per week. The weekly training time among the recreational athletes was about five to eight hours. Additional inclusion criteria were: ≥18 years of age and a positive SARS-CoV-2 PCR test or antibody detection with additional typical symptoms. The exclusion criteria were: acute or chronic illness that precluded a planned physical examination, acute SARS-CoV-2 infection, refusal of peripheral venous blood sampling, inadequate German language skills, and withdrawal from study participation. Athletes provided written informed consent after being instructed of the study procedures (30). The study was conducted in accordance with the Declaration of Helsinki and approved by the local ethics committee of the University of Ulm (EK 408/20).
2.2. Clinical evaluation of the participants
All athletes underwent a clinical evaluation that included a medical history and physical examination, 12-lead electrocardiogram (ECG), transthoracic echocardiography (TTE) including determination of left ventricular global longitudinal strain (LV GLS), and cardiopulmonary exercise testing (CPET). To examine the long-term course of echocardiographic and CPET findings, participants were invited for a follow-up three months after the initial clinical evaluation.
2.3. Transthoracic echocardiography
Transthoracic examination was performed using an EPIQ 7 ultrasound system with a phased-array probe X5-1 (Philips GmbH, Hamburg, Germany). The following parameters were collected: left ventricular internal diameter end diastole (LVIDd) and end systole (LVIDs). LVIDd (LVIDd/BSA) and LVIDs (LVIDs/BSA) were indexed to body surface area (BSA), left ventricular ejection fraction (LV-EF by biplane LV planimetry by Simpson), fractional shortening (FS), left ventricular mass (LV mass), LV mass/BSA, stroke volume (SV), septal thickness [= interventricular septal end diastole (IVSd)] and posterior wall thickness [= left ventricular posterior wall end diastole (LVPWd)] and right ventricle longitudinal function by tricuspid annular plane systolic excursion (TAPSE). Diastolic function was characterized by E/A ratio, E/Élateral ratio, E/E'medial ratio and deceleration time (Dec Time) (31, 32).
2.4. Strain measurements
LV GLS was determined offline using TomTec postprocessing software (2D Cardiac Performance Analysis, TomTec Imaging Systems, Unterschleissheim, Germany) by an examiner who was blinded to patient history. LV GLS was obtained in apical four-chamber, two-chamber and long-axis views in the apical, midline, and basal segments (33). The endocardial contour was manually adjusted. Regardless of provider or clinical covariates, a LV GLS ≥−16% (less negative) was considered abnormal (34). A selection of 20 images was reviewed a second time by the same blinded investigator and by a second blinded investigator to determine intrarater and interrater reliability.
2.5. Cardiopulmonary exercise testing (CPET)
CPET was performed on a cycle ergometer (Excalibur Sport, LODE B.V., Groningen, The Netherlands) using a breath-by-breath gas analysis system (Ergostik, Geratherm Respiratory, Bad Kissingen, Germany). All athletes performed an incremental exercise test. An individually adjusted ramp protocol was chosen according to age, gender, weight and estimated fitness level to exhaust subjects within 8–12 min (35). A 12 lead ECG recording system (Cardiopart 12 Blue/Blue-P, AMEDTEC Medizintechnik Aue GmbH, Aue, Germany) was used. All CPETs were evaluated by the same investigator according to Wasserman et al. (35) and Clinical Recommendations for Cardiopulmonary Exercise Testing Data Assessment in Specific Patient Populations (36). The following variables were measured or calculated: maximum power and predicted maximum power, respiratory oxygen uptake at first ventilatory threshold (VO2@VT1/kg) and peak respiratory oxygen uptake (peak VO2/kg), peak respiratory exchange rate (RER), heart rate (HR) at VT1 (HR@VT1), HR at peak respiratory oxygen uptake (HR@peak VO2) and predicted HR at peak respiratory oxygen uptake (predicted HR@peak VO2), peak oxygen pulse (peak O2/HR) and predicted peak oxygen pulse and the ventilation/volume of CO2 slope (VE/VCO2 slope).
2.6. Statistical analysis
Statistical analyses were performed using R Project for Statistical Computing version 4.1.1 (RRID:SCR_001905) (37) and GraphPad Prism 9 (Version 9.4.1, GraphPad Software Inc., California, USA, RRID:SCR_002798). The descriptive data are presented as median and interquartile ranges (IQR). Assumptions for linear regression were visually verified using residual, QQ plots, and histograms. For distribution analysis, Shapiro-Wilk test was used. Correlations between LV GLS and age and BMI were analyzed using Spearman's ρ. The change of cardiac and performance parameters over the three month period was assessed using robust linear mixed effects regression models (38) separately controlling for possible confounding variables (BMI, age, sex, HR, systolic and diastolic blood pressure). A p-value of <0.05 was considered significant. We performed an additional exploratory analysis in a subsample of six athletes with a GLS ≥−16%, defined as reduced GLS. Comparisons were made with a paired t-test if the distribution was normal, otherwise a Wilcoxon signed-rank test was used.
3. Results
3.1. Cohort characteristics
A total of 96 athletes (mean age 33.15 ± 12.40 years, 53 male) were included in the statistical analysis. The sports practiced were divided as follows: 61% endurance sports, 13% strength and combat sports, 21% team sports and 5% technical sports (Table 1). Symptoms reported during COVID-19 infection were standard clinical symptoms of viral infection: fever (39%), cough (46%), rhinorrhea (52%), sore throat (45%), resting dyspnea (21%) or exertional dyspnea (34%), and subjectively perceived reduction in performance (39%) compared with maximal performance before COVID-19. Cardiac symptoms were observed in the form of palpitations (21%), chest pain (21%), increased resting heart rate (26%) or exertional dyspnea after COVID-19 (30%) (Table 2).
3.2. Echocardiographic parameters
LV GLS improved significantly between t0 and t1 (t0 (−18.82 ± 2.02 vs. t1 −19.46 ± 2.05, p < 0.001). All echocardiographic parameters were within the normal range. There were no significant differences between the study time points (Table 3, Supplementary Table S1). Significant changes in LV GLS persisted over time even after adjustment for confounding variables (age, sex, heart rate, BMI, systolic or diastolic blood pressure) (Supplementary Table S2). Intrarater and interrater reliability with respect to the LV GLS measure showed high agreement (intrarater: 0.892 [95%CI, 0.593–0.973]; interrater: 0.794 [95%CI, 0.159–0.949]).
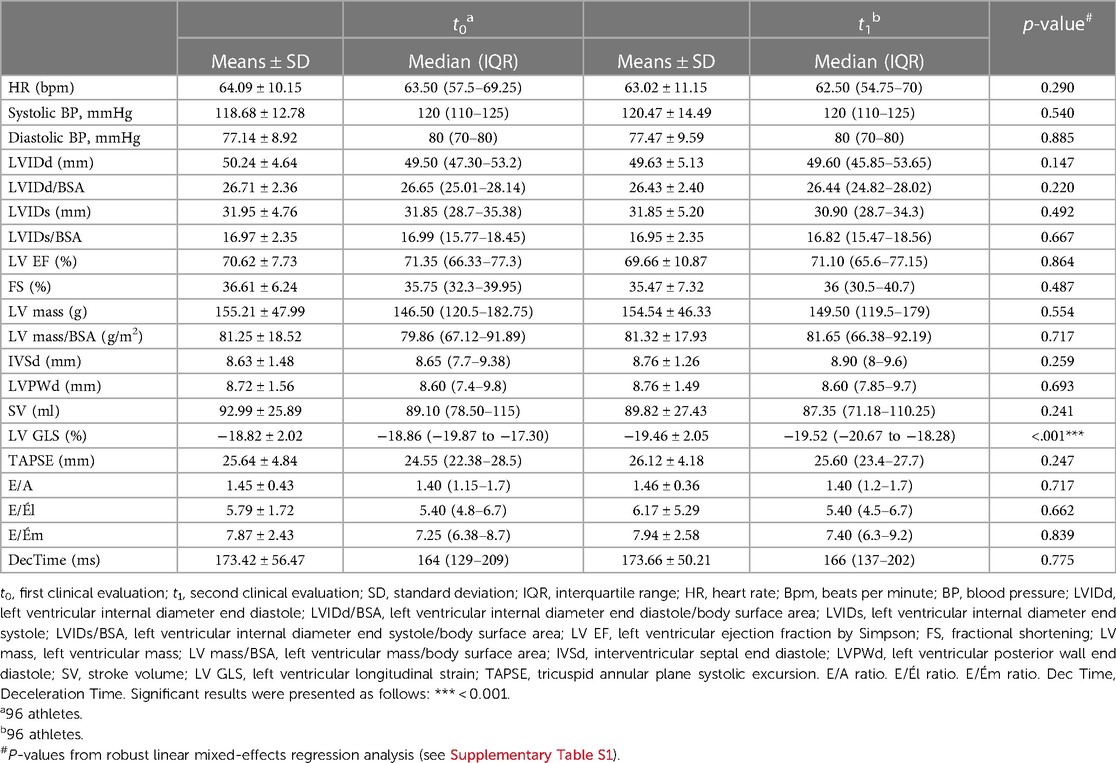
Table 3. Echocardiographic parameters at study time t0 and t1 presented as means and standard deviation or median and IQR.
3.3. Cardiopulmonary exercise testing (CPET)
Maximum power was significant higher at t1 than at t0 (t0 283.17 ± 83.20 Watt vs. t1 286.24 ± 85.22 Watt, p = 0.009). There were no significant differences between t0 and t1 in performance parameters such as peak oxygen pulse or VE/VCO2 slope but there was a trend toward increased peak oxygen uptake (t0 36.82 ± 11.14 ml/min/kg vs. t1 38.68 ± 10.26 ml/min/kg, p = 0.069) (Table 4, Supplementary Table S1). Baseline heart rate was 64.09 ± 10.15 bpm at t0 and 63.02 ± 11.15 bpm at t1 and was not significantly different. Heart rate increased only slightly but not significantly (t0 170.70 ± 17.50 bpm vs. t1 172.63 ± 14.10 bpm, p = 0.480). Athletes achieved the same maximal effort at both time points (RER 1.22 vs. 1.22, p = 0.424) (Table 4).
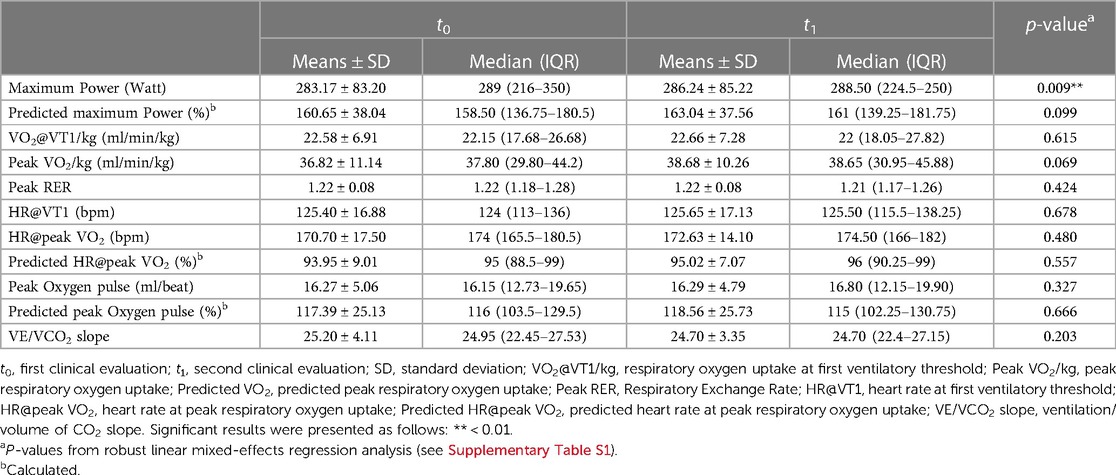
Table 4. CPET parameters at study time t0 and t1 presented as means and standard deviation or median and IQR.
3.4. Correlation of LV GLS with performance parameters
LV GLS correlates inversely with RER (−0.316 [−0.501; 0.102], S = 129,938.15, p = 0.027) (Supplementary Table S3). This means that athletes with inadequate exercise intolerance also have the worse (more positive) GLS values. LV GLS did not correlate with other performance parameters such as oxygen uptake, peak oxygen pulse or VE/VCO2 slope (Supplementary Table S3).
3.5. Subgroup analysis of athletes with reduced LV GLS
Six athletes (mean age 37 years, three male) had a reduced LV GLS of ≥ −16.0% (less negative) (Figure 1). In four athletes the LV GLS normalized and in two athletes it remained constantly slightly reduced. Subacute myocarditis was detected by MRI in a female athlete with reduced LV GLS. LV EF improved here (51.3% vs. 74%), and performance was identical at both examination time points. An increase in peak VO2 was seen in two cases. In three cases it remained the same and in one athlete there was a decrease in peak VO2. In one female athlete (red points in Figure 1) out of 96 studied, a LV GLS previously classified as normal, increased without evidence of heart disease or performance impairment. For all parameters shown in Figure 1, there was no significant difference between the two study time points.
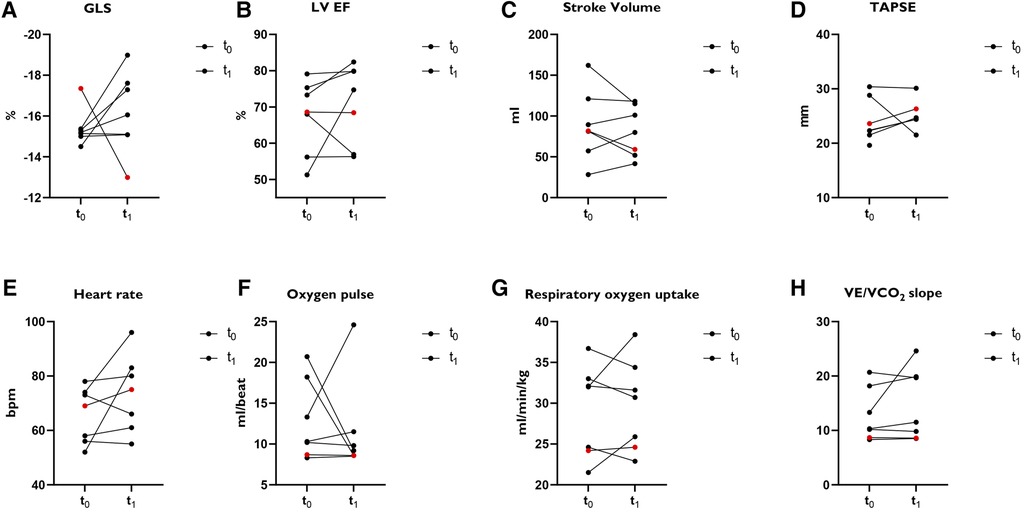
Figure 1. Subanalysis of athletes (N = 7) with LV GLS ≥ −16.0%. (A) GLS: Global longitudinal strain. (B) LV EF: left ventricular ejection fraction. (C) Stroke volume. (D) TAPSE: tricuspid annular plane systolic excursion. (E) Heart rate. Bpm = beats per minute. (F) Oxygen pulse. (G) Oxygen uptake. (H) VE/VO2 slope. Red points: Female athlete with a normal classified GLS at t0 and increased GLS at t1 without signs of heart disease or performance impairment.
4. Discussion
Whether a reduction in LV GLS also leads to reduced performance in athletes after SARS-CoV-2 infection has been insufficiently investigated. We observed significant improvement in LV GLS approximately five months after COVID-19 compared to baseline at a median of two months after COVID-19, while we did not find any correlation between LV GLS and performance parameters, with the exception of RER.
4.1. Development of LV GLS
To our knowledge, this is the first study to investigate the course of LV GLS and the impact on performance in athletes after COVID-19. Our preliminary study results indicate a mild myocardial involvement in the form of reduced LV GLS in athletes after COVID-19 compared with healthy athletes (26). However, to date, there are no clear results and evidence that cardiac involvement occurs in athletes after COVID-19, and long-term observations in athletes are scarce (24, 25). Hospitalized patients without athletic background did not show significant improvement in LV-GLS at two months (27) or three months (28) or after acute infection: In the study by Baruch et al., 80 hospitalized patients (mean age 57.7 ± 14.9 years, 54 male) showed no significant difference in LV GLS 88.2 ± 33 days after baseline assessment, but 20 patients (25%) still had abnormal LV GLS (28). Fifty-two patients had moderate disease (pneumonia with a grade of ≥94% without oxygenation) and 28 patients had a severe disease course, so it is obvious that the included study population does not correspond to that of athletes in our work. In addition, the patients were significantly older and had previous cardiopulmonary diseases. Obesity, which may affect left ventricular function as measured by LV EF and LV GLS, was present in 19 patients (23.8%) (40–42).
Similar results were seen in the Danish prospective longitudinal cohort study ECHOVID-19 by Lassen et al. (27). Whereas right ventricular function improved after a median of 77 days, in contrast, LV GLS did not improve significantly. In addition, recovered COVID-19 patients had significantly lower LV GLS compared with age- and sex-matched controls from individuals participating in the Copenhagen City Heart Study (43). These 91 patients (mean age 63 ± 12 years, 54 male) were elderly and had heart failure or ischemic heart disease (11%) and hypertension (48%) and, most importantly, were also not athletes. LV GLS has been shown to be significantly reduced in patients with hypertension compared with normotensive control subjects (44) because blood pressure correlates with LV GLS (45, 46).
The findings of Baruch et al. and Lassen et al. lead in a different direction than our results, but this may be due to differences in the population studied (age, preexisting conditions, disease course). Patients who required hospitalization may have a higher prevalence of undetected subclinical heart disease than COVID-19 patients who were not hospitalized. Prolonged follow-up examinations may be needed here to show possible recovery. However, in the follow-up study by Karagodin et al., improvements in LV GLS were noted in patients with impaired baseline function (29). Overall, in this study, there was no significant change in LV GLS over time (230 ± 115 days) in 153 hospitalized patients (median age 57, 80 male). The improvement in LV GLS in patients with impaired baseline function may reflect recovery from acute myocardial injury in severely ill patients (31% in intensive care unit, 16.3% with ventilation, 8.5% with hemodynamic support). Our non-hospitalized athletes described mild courses with standard clinical symptoms of viral infection such as fever, cough, rhinorrhea, sore throat, dyspnea at rest or on exertion, and subjectively perceived decrease in performance (4). Cardiac symptoms such as palpitations, chest pain, and increased resting heart rate occurred in only 21%–26%. Recovery appears to be faster in healthy subjects without pre-existing myocardial dysfunction, which may be reflected in improvement of LV GLS. In general, highly trained athletes are known to have normal, albeit slightly lower, LV GLS and strain rate parameters compared to untrained control subjects (47, 48). Thus, there seems not to be a difference in baseline LV GLS here with that in the long-term studies presented. It still remains unknown what are the origins of the decreased LV myocardial function. It may be a consequence of direct cardiac injury from SARS-CoV-2 infection or a secondary consequence of systemic inflammation, or a combination of both (1, 3). Of course, ischemic injury caused by cardiac microvascular dysfunction cannot be excluded (49).
4.2. LV GLS and performance parameters
To date, no study has examined the relationship between LV GLS and physical performance in athletes recovering from COVID-19. However, in patients with ischemic heart disease (IHD), LV GLS is associated with decreased maximal oxygen uptake, which is an independent risk factor for adverse cardiovascular events (50). In addition, LV GLS correlated independently with peak VO2 in studies of patients with reduced (rEF) and pEF and was superior to LV EF in identifying patients with reduced exercise capacity (51–53).
In a study by Shimoni et al, patients were examined 57 (27–100) days after COVID-19. Subclinical impairment of LV function was shown to correlate with lower physical performance and duration, but no direct cause-effect relationship could be demonstrated (54). The correlation of LV GLS and physical performance could be due to persistent myocardial damage, and perhaps also to significant age differences (mean age 48 ± 12 years, 87 men) compared with our study group. In our study population of atheltes, LV GLS was within the normal range at t0 and t1, and we assume normal cardiac function with only mild myocardial involvement. Therefore, without the exception of RER, we could not demonstrate a correlation with performance parameters. The inverse correlation of GLS with RER indicates insufficient load intolerance at lower GLS values. There is no study to date that reflects this fact. However, in previous studies, submaximal exercise was observed in severe initial disease course (55) or as a sign of possible deconditioning (56) in Post-COVID patients. The number of athletes with reduced LV GLS (≥ −16.0%) may be too small to demonstrate statistical significance. Whether LV GLS correlates independently of COVID-19 with performance parameters in athletes in general has not been investigated to date. However, this could provide interesting additional information about cardiac adaptation processes in competitive as well as recreational athletes and could be integrated into diagnostics of the annual sports medical screening or return-to-sport examination in the future.
4.3. Development of spiroergometric parameters
Maximum power increased significantly from t0 to t1 and there is a trend toward increased peak oxygen uptake. It cannot be ruled out that a break in training in the context of SARS-CoV-2 infection is causing poorer performance, which subsequently improves during the course. Improvement in performance could also be explained by the athletes' increasing recovery and predominantly being symptom-free at t1. The oxygen pulse, reflecting the maximal aerobic capacity, does not change significantly and heart rate increases only slightly but not significantly. These results emphasize that cardiac function does not appear to be impaired. Athletes achieved the same maximal effort at both time points (RER 1.22 vs. 1.22, p = 0.424). Similarly, in subgroup analysis of six athletes with probable myocardial dysfunction (GLS ≥ −16.0%), heart rate, LV GLS, peak oxygen pulse, or maximal oxygen uptake did not differ significantly between study time points.
This is broadly consistent with results from Komici et al, who found no decreased physical performance or impairment in pulmonary and cardiovascular function during the early recovery period (10–30 days) after COVID-19 in 24 competitive soccer players (mean age 23.5 years, 24 male) compared to healthy control athletes. However, LV GLS was not examined here (57). Two previously published studies showed an improvement in symptoms after COVID with corresponding improvements in CPET parameters over a longer observation period (58, 59). The main difference between the study by Moulson et al. and ours was that it included athletes with persistent cardiopulmonary symptoms, thus presenting a highly selected subgroup of athletes after COVID-19. These athletes were significantly more likely to report symptoms of dyspnea (76% vs. 21%), exercise intolerance (76% vs. 39%), chest pain (71% vs. 21%), and palpitations (57% vs. 21%) than the athletes we studied (who had partially recovered and were symptom-free), which may explain initially worse performance and subsequent improvement (58). Due to the different study populations and designs, there are no consensus data available so far, which limits the comparability between current studies.
4.4. Strengths and limitations
This presented study is limited by the longitudinal design, as strain values and performance parameters of athletes from periods prior to COVID-19 are not available. Follow-up examinations were performed after five months on the assumption that this period was sufficiently long to detect recovery of cardiac function. For possible variables influencing GLS, such as age, sex, heart rate, BMI, systolic or diastolic blood pressure, we performed multivariate linear regression. Significant changes in LV GLS persisted over the observation period. Although the LV GLS determination can be software and investigator experience dependent, our results show high intrarater and interrater reliability. On the one hand, the clearly defined population of study participants, consisting of athletes, limits the generalizability of the results to the general population. But, on the other hand, it allows an assessment of a specific group. It should also be emphasized that we achieved a meaningful case number of athletes for a single-centre study. Finally, although an association between COVID-19 and the occurrence of pathological examination findings up to myocarditis is suggested, direct evidence is still lacking.
4.5. Conclusion
We observed significant improvements in LV GLS approximately five months after COVID-19 compared with baseline at a median of two months after COVID-19. Therefore, we assume that the significant LV-GLS differences reflect possible mild myocardial involvement during or shortly after COVID-19. Except for RER, we did not find a correlation between LV GLS and performance parameters. This could indicate that mild cardiac dysfunction in athletes with mild disease course does not necessarily contribute to decreased performance after COVID-19. However, an inverse correlation between GLS and RER seems to indicate insufficient load intolerance at lower GLS values. Here, further studies on the development of GLS in athletes or in the general population with moderate and severe disease courses would be informative as well as the comparison of pre-COVID-19 with post-COVID-19 echocardiography to evaluate the effects of COVID-19 on cardiac function.
Data availability statement
The original contributions presented in the study are included in the article/Supplementary Material, further inquiries can be directed to the corresponding author.
Ethics statement
The studies involving humans were approved by Local Ethics Committee of the University of Ulm (EK 408/20). The studies were conducted in accordance with the local legislation and institutional requirements. The participants provided their written informed consent to participate in this study.
Author contributions
Conceptualization, JS and JMS; Data curation, JS and SV; Formal analysis, JS and LM; Funding acquisition, JMS; Investigation, JS and SV; Methodology, JS, LM, DAB, JKe, SV, and JMS; Project administration, JMS; Resources, JS; Software, JS and LM; Supervision, JMS; Validation, JS, LM, DAB, and JMS; Visualization, JS and LM; Writing—original draft, JS; Writing—review & editing, JS, DAB, LM, JKi, JKe, SV, and JMS All authors have read and agreed to the published version of the manuscript. All authors contributed to the article and approved the submitted version.
Funding
This work was supported primarily by the University Hospital Ulm and funds from the German Federal Institute for Sport Science, Cologne by resolution of the German Bundestag [ZMVI4-070106/20-23].
Conflict of interest
The authors declare that the research was conducted in the absence of any commercial or financial relationships that could be construed as a potential conflict of interest.
Publisher's note
All claims expressed in this article are solely those of the authors and do not necessarily represent those of their affiliated organizations, or those of the publisher, the editors and the reviewers. Any product that may be evaluated in this article, or claim that may be made by its manufacturer, is not guaranteed or endorsed by the publisher.
Supplementary material
The Supplementary Material for this article can be found online at: https://www.frontiersin.org/articles/10.3389/fcvm.2023.1240278/full#supplementary-material
References
1. Akhmerov A, Marban E. COVID-19 and the heart. Circ Res. (2020) 126(10):1443–55. doi: 10.1161/CIRCRESAHA.120.317055
2. Huang C, Wang Y, Li X, Ren L, Zhao J, Hu Y, et al. Clinical features of patients infected with 2019 novel coronavirus in Wuhan, China. Lancet. (2020) 395(10223):497–506. doi: 10.1016/S0140-6736(20)30183-5
3. Zheng YY, Ma YT, Zhang JY, Xie X. COVID-19 and the cardiovascular system. Nat Rev Cardiol. (2020) 17(5):259–60. doi: 10.1038/s41569-020-0360-5
4. Krzywanski J, Mikulski T, Krysztofiak H, Pokrywka A, Mlynczak M, Malek LA, et al. Elite athletes with COVID-19—predictors of the course of disease. J Sci Med Sport. (2022) 25(1):9–14. doi: 10.1016/j.jsams.2021.07.003
5. Rajpal S, Tong MS, Borchers J, Zareba KM, Obarski TP, Simonetti OP, et al. Cardiovascular magnetic resonance findings in competitive athletes recovering from COVID-19 infection. JAMA Cardiol. (2021) 6(1):116–8. doi: 10.1001/jamacardio.2020.4916. Erratum in: JAMA Cardiol. (2021) 6(1):123.32915194
6. Schumacher YO, Tabben M, Hassoun K, Al Marwani A, Al Hussein I, Coyle P, et al. Resuming professional football (soccer) during the COVID-19 pandemic in a country with high infection rates: a prospective cohort study. Br J Sports Med. (2021) 55(19):1092–8. doi: 10.1136/bjsports-2020-103724
7. Clark DE, Parikh A, Dendy JM, Diamond AB, George-Durrett K, Fish FA, et al. COVID-19 Myocardial pathology evaluation in athletes with cardiac magnetic resonance (COMPETE CMR). Circulation. (2021) 143(6):609–12. doi: 10.1161/CIRCULATIONAHA.120.052573
8. Moulson N, Petek BJ, Drezner JA, Harmon KG, Kliethermes SA, Patel MR, et al. SARS-CoV-2 cardiac involvement in young competitive athletes. Circulation. (2021) 144(4):256–66. doi: 10.1161/CIRCULATIONAHA.121.054824
9. Martinez MW, Tucker AM, Bloom OJ, Green G, DiFiori JP, Solomon G, et al. Prevalence of inflammatory heart disease among professional athletes with prior COVID-19 infection who received systematic return-to-play cardiac screening. JAMA Cardiol. (2021) 6(7):745–52. doi: 10.1001/jamacardio.2021.0565
10. Steinacker JM*, Schellenberg J*, Bloch W, Deibert P, Friedmann-Bette B, Grim C, et al. Recommendations for return-to-sport after COVID-19: expert consensus. DZSM. (2022) 73(4):127–36. doi: 10.5960/dzsm.2022.532
11. Wilson MG, Hull JH, Rogers J, Pollock N, Dodd M, Haines J, et al. Cardiorespiratory considerations for return-to-play in elite athletes after COVID-19 infection: a practical guide for sport and exercise medicine physicians. Br J Sports Med. (2020) 54(19):1157–61. doi: 10.1136/bjsports-2020-102710
12. Halle M, Bloch W, Niess AM, Predel HG, Reinsberger C, Scharhag J, et al. Exercise and sports after COVID-19-guidance from a clinical perspective. Transl Sports Med. (2021) 4(3):310–8. doi: 10.1002/tsm2.247
13. Blessberger H, Binder T. Two dimensional speckle tracking echocardiography: clinical applications. Heart. (2010) 96(24):2032–40. doi: 10.1136/hrt.2010.199885
14. Smiseth OA, Torp H, Opdahl A, Haugaa KH, Urheim S. Myocardial strain imaging: how useful is it in clinical decision making? Eur Heart J. (2016) 37(15):1196–207. doi: 10.1093/eurheartj/ehv529
15. Biering-Sorensen T, Biering-Sorensen SR, Olsen FJ, Sengelov M, Jorgensen PG, Mogelvang R, et al. Global longitudinal strain by echocardiography predicts long-term risk of cardiovascular morbidity and mortality in a low-risk general population: the Copenhagen City Heart Study. Circ Cardiovasc Imaging. (2017) 10(3):e005521. doi: 10.1161/CIRCIMAGING.116.005521
16. Croft LB, Krishnamoorthy P, Ro R, Anastasius M, Zhao W, Buckley S, et al. Abnormal left ventricular global longitudinal strain by speckle tracking echocardiography in COVID-19 patients. Future Cardiol. (2021) 17(4):655–61. doi: 10.2217/fca-2020-0121
17. Stobe S, Richter S, Seige M, Stehr S, Laufs U, Hagendorff A. Echocardiographic characteristics of patients with SARS-CoV-2 infection. Clin Res Cardiol. (2020) 109(12):1549–66. doi: 10.1007/s00392-020-01727-5
18. Li R, Wang H, Ma F, Cui GL, Peng LY, Li CZ, et al. Widespread myocardial dysfunction in COVID-19 patients detected by myocardial strain imaging using 2-D speckle-tracking echocardiography. Acta Pharmacol Sin. (2021) 42(10):1567–74. doi: 10.1038/s41401-020-00595-z
19. Minhas AS, Gilotra NA, Goerlich E, Metkus T, Garibaldi BT, Sharma G, et al. Myocardial work efficiency, a novel measure of myocardial dysfunction, is reduced in COVID-19 patients and associated with in-hospital mortality. Front Cardiovasc Med. (2021) 8:667721. doi: 10.3389/fcvm.2021.667721
20. Baum P, Do L, Deterding L, Lier J, Kunis I, Saur D, et al. Cardiac function in relation to functional status and fatigue in patients with post-COVID syndrome. Sci Rep. (2022) 12(1):19575. doi: 10.1038/s41598-022-24038-3
21. Mahajan S, Kunal S, Shah B, Garg S, Palleda GM, Bansal A, et al. Left ventricular global longitudinal strain in COVID-19 recovered patients. Echocardiography. (2021) 38(10):1722–30. doi: 10.1111/echo.15199
22. Kujur PP, Jhala M, Bhondve A, Lanjewar C, Matta R, Deshmukh H. Left ventricular global longitudinal strain imaging in identifying subclinical myocardial dysfunction among COVID-19 survivors. Indian Heart J. (2022) 74(1):51–5. doi: 10.1016/j.ihj.2021.12.007
23. Oikonomou E, Lampsas S, Theofilis P, Souvaliotis N, Papamikroulis GA, Katsarou O, et al. Impaired left ventricular deformation and ventricular-arterial coupling in post-COVID-19: association with autonomic dysregulation. Heart Vessels. (2023) 38(3):381–93. doi: 10.1007/s00380-022-02180-2
24. Lakatos BK, Tokodi M, Fabian A, Ladanyi Z, Vago H, Szabo L, et al. Frequent constriction-like echocardiographic findings in elite athletes following mild COVID-19: a propensity score-matched analysis. Front Cardiovasc Med. (2021) 8:760651. doi: 10.3389/fcvm.2021.760651
25. Fikenzer S, Kogel A, Pietsch C, Lavall D, Stobe S, Rudolph U, et al. SARS-CoV2 infection: functional and morphological cardiopulmonary changes in elite handball players. Sci Rep. (2021) 11(1):17798. doi: 10.1038/s41598-021-97120-x
26. Schellenberg J, Ahathaller M, Matits L, Kirsten J, Kersten J, Steinacker JM. Left ventricular global longitudinal strain as a parameter of mild myocardial dysfunction in athletes after COVID-19. J Cardiovasc Dev Dis. (2023) 10(5):189. doi: 10.3390/jcdd10050189
27. Lassen MCH, Skaarup KG, Lind JN, Alhakak AS, Sengelov M, Nielsen AB, et al. Recovery of cardiac function following COVID-19—eCHOVID-19: a prospective longitudinal cohort study. Eur J Heart Fail. (2021) 23(11):1903–12. doi: 10.1002/ejhf.2347
28. Baruch G, Rothschild E, Sadon S, Szekely Y, Lichter Y, Kaplan A, et al. Evolution of right and left ventricle routine and speckle-tracking echocardiography in patients recovering from coronavirus disease 2019: a longitudinal study. Eur Heart J Cardiovasc Imaging. (2022) 23(8):1055–65. doi: 10.1093/ehjci/jeab190
29. Karagodin I, Singulane CC, Descamps T, Woodward GM, Xie M, Tucay ES, et al. Ventricular changes in patients with acute COVID-19 infection: follow-up of the world alliance societies of echocardiography (WASE-COVID) study. J Am Soc Echocardiogr. (2022) 35(3):295–304. doi: 10.1016/j.echo.2021.10.015
30. Niess AM, Widmann M, Gaidai R, Golz C, Schubert I, Castillo K, et al. COVID-19 in German competitive sports: protocol for a prospective multicenter cohort study (CoSmo-S). Int J Public Health. (2022) 67:1604414. doi: 10.3389/ijph.2022.1604414
31. Lang RM, Badano LP, Mor-Avi V, Afilalo J, Armstrong A, Ernande L, et al. Recommendations for cardiac chamber quantification by echocardiography in adults: an update from the American Society of Echocardiography and the European Association of Cardiovascular Imaging. Eur Heart J Cardiovasc Imaging. (2015) 16(3):233–70. doi: 10.1093/ehjci/jev014
32. Quinones MA, Otto CM, Stoddard M, Waggoner A, Zoghbi WA, Doppler Quantification Task Force of the Net al.. Recommendations for quantification of doppler echocardiography: a report from the doppler quantification task force of the nomenclature and standards committee of the American Society of Echocardiography. J Am Soc Echocardiogr. 2002;15(2):167–84. doi: 10.1067/mje.2002.120202
33. Voigt JU, Pedrizzetti G, Lysyansky P, Marwick TH, Houle H, Baumann R, et al. Definitions for a common standard for 2D speckle tracking echocardiography: consensus document of the EACVI/ASE/industry task force to standardize deformation imaging. J Am Soc Echocardiogr. (2015) 28(2):183–93. doi: 10.1016/j.echo.2014.11.003
34. D'Elia N, Caselli S, Kosmala W, Lancellotti P, Morris D, Muraru D, et al. Normal global longitudinal strain: an individual patient meta-analysis. JACC Cardiovasc Imaging. (2020) 13(1 Pt 1):167–9. doi: 10.1016/j.jcmg.2019.07.020
35. Wassermann K, Pothoff G, Subbe C, Bahra J, Hilger HH. Air trapping and cardiopulmonary exercise testing in patients with mild to moderate bronchial obstruction: clinical role of trapped gas measurements. Z Kardiol. (1994) 83(Suppl 3):149–54.7941663
36. Guazzi M, Arena R, Halle M, Piepoli MF, Myers J, Lavie CJ. 2016 Focused update: clinical recommendations for cardiopulmonary exercise testing data assessment in specific patient populations. Circulation. (2016) 133(24):e694–711. doi: 10.1161/CIR.0000000000000406
37. R-Core-Team. R: A language and environment for statistical computing.: R Foundation for Statistical Computing; 2022.
38. Koller M. Robustlmm: an R package for robust estimation of linear mixed-effects models. J Stat Softw. (2016) 75(6):1–24. doi: 10.18637/jss.v075.i06
39. Pelliccia A, Sharma S, Gati S, Back M, Borjesson M, Caselli S, et al. 2020 ESC guidelines on sports cardiology and exercise in patients with cardiovascular disease. Eur Heart J). (2021) 42(1):17–96. doi: 10.1093/eurheartj/ehaa605. Erratum in: Eur Heart J. (2021) 42(5):548–49.
40. Blomstrand P, Sjoblom P, Nilsson M, Wijkman M, Engvall M, Lanne T, et al. Overweight and obesity impair left ventricular systolic function as measured by left ventricular ejection fraction and global longitudinal strain. Cardiovasc Diabetol. (2018) 17(1):113. doi: 10.1186/s12933-018-0756-2
41. Arenas IA, Podesta CA, Issa O, Lin J, Brenes JC. Myocardial longitudinal strain, fitness, and heart failure risk factors in young adults. Echocardiography. (2020) 37(3):404–11. doi: 10.1111/echo.14613
42. Siurana JM, Ventura PS, Yeste D, Riaza-Martin L, Arciniegas L, Clemente M, et al. Myocardial geometry and dysfunction in morbidly obese adolescents (BMI 35-40 kg/m(2)). Am J Cardiol. (2021) 157:128–34. doi: 10.1016/j.amjcard.2021.07.026
43. Skaarup KG, Lassen MCH, Johansen ND, Olsen FJ, Lind JN, Jorgensen PG, et al. Age- and sex-based normal values of layer-specific longitudinal and circumferential strain by speckle tracking echocardiography: the Copenhagen City Heart Study. Eur Heart J Cardiovasc Imaging. (2022) 23(5):629–40. doi: 10.1093/ehjci/jeab032
44. Tadic M, Majstorovic A, Pencic B, Ivanovic B, Neskovic A, Badano L, et al. The impact of high-normal blood pressure on left ventricular mechanics: a three-dimensional and speckle tracking echocardiography study. Int J Cardiovasc Imaging. (2014) 30(4):699–711. doi: 10.1007/s10554-014-0382-3
45. Galderisi M, Esposito R, Schiano-Lomoriello V, Santoro A, Ippolito R, Schiattarella P, et al. Correlates of global area strain in native hypertensive patients: a three-dimensional speckle-tracking echocardiography study. Eur Heart J Cardiovasc Imaging. (2012) 13(9):730–8. doi: 10.1093/ehjci/jes026
46. Saghir M, Areces M, Makan M. Strain rate imaging differentiates hypertensive cardiac hypertrophy from physiologic cardiac hypertrophy (athlete’s heart). J Am Soc Echocardiogr. (2007) 20(2):151–7. doi: 10.1016/j.echo.2006.08.006
47. Caselli S, Montesanti D, Autore C, Di Paolo FM, Pisicchio C, Squeo MR, et al. Patterns of left ventricular longitudinal strain and strain rate in Olympic athletes. J Am Soc Echocardiogr. (2015) 28(2):245–53. doi: 10.1016/j.echo.2014.10.010
48. D’Ascenzi F, Solari M, Mazzolai M, Cameli M, Lisi M, Andrei V, et al. Two-dimensional and three-dimensional left ventricular deformation analysis: a study in competitive athletes. Int J Cardiovasc Imaging. (2016) 32(12):1697–705. doi: 10.1007/s10554-016-0961-6
49. Fox SE, Lameira FS, Rinker EB, Vander Heide RS. Cardiac endotheliitis and multisystem inflammatory syndrome after COVID-19. Ann Intern Med. (2020) 173(12):1025–7. doi: 10.7326/L20-0882
50. Zhang S, Liu Y, Jiang L, Wang Z, Liu W, Zuo H. Diagnostic accuracy of global longitudinal strain for detecting exercise intolerance in patients with ischemic heart disease. J Cardiovasc Dev Dis. (2023) 10(1):10. doi: 10.3390/jcdd10010010
51. Maia RJC, Brandao SCS, Leite J, Parente GB, Pinheiro F, Araújo BTS, et al. Global longitudinal strain predicts poor functional capacity in patients with systolic heart failure. Arq Bras Cardiol. (2019) 113(2):188–94. doi: 10.5935/abc.20190119
52. Hasselberg NE, Haugaa KH, Sarvari SI, Gullestad L, Andreassen AK, Smiseth OA, et al. Left ventricular global longitudinal strain is associated with exercise capacity in failing hearts with preserved and reduced ejection fraction. Eur Heart J Cardiovasc Imaging. (2015) 16(2):217–24. doi: 10.1093/ehjci/jeu277
53. Li M, Lu Y, Fang C, Zhang X. Correlation between myocardial deformation on three-dimensional speckle tracking echocardiography and cardiopulmonary exercise testing. Echocardiography. (2017) 34(11):1640–8. doi: 10.1111/echo.13675
54. Shimoni O, Korenfeld R, Goland S, Meledin V, Haberman D, George J, et al. Subclinical myocardial dysfunction in patients recovered from COVID-19 disease: correlation with exercise capacity. Biology (Basel). (2021) 10(11):1201. doi: 10.3390/biology10111201
55. Romero-Ortuno R, Jennings G, Xue F, Duggan E, Gormley J, Monaghan A. Predictors of submaximal exercise test attainment in adults reporting long COVID symptoms. J Clin Med. (2022) 11(9):2376. doi: 10.3390/jcm11092376
56. Rinaldo RF, Mondoni M, Parazzini EM, Pitari F, Brambilla E, Luraschi S, et al. Deconditioning as main mechanism of impaired exercise response in COVID-19 survivors. Eur Respir J. (2021) 58(2):2100870. doi: 10.1183/13993003.00870-2021
57. Komici K, Bianco A, Perrotta F, Dello Iacono A, Bencivenga L, D’Agnano V, et al. Clinical characteristics, exercise capacity and pulmonary function in post-COVID-19 competitive athletes. J Clin Med. (2021) 10(14):3053. doi: 10.3390/jcm10143053
58. Moulson N, Gustus SK, Scirica C, Petek BJ, Vanatta C, Churchill TW, et al. Diagnostic evaluation and cardiopulmonary exercise test findings in young athletes with persistent symptoms following COVID-19. Br J Sports Med. (2022) 56:927–32. doi: 10.1136/bjsports-2021-105157
Keywords: sport, COVID-19, speckle tracking echocardiography, performance, CPET
Citation: Schellenberg J, Matits L, Bizjak DA, Kersten J, Kirsten J, Vollrath S and Steinacker JM (2023) Assessment of myocardial function and cardiac performance using left ventricular global longitudinal strain in athletes after COVID-19: a follow-up study. Front. Cardiovasc. Med. 10:1240278. doi: 10.3389/fcvm.2023.1240278
Received: 14 June 2023; Accepted: 25 September 2023;
Published: 9 October 2023.
Edited by:
Sanjeev Bhattacharyya, Barts Heart Centre, United KingdomReviewed by:
Francesco Bianco, Azienda Ospedaliero Universitaria Ospedali Riuniti, ItalyNicole Panhuyzen-Goedkoop, Amsterdam University Medical Center, Netherlands
© 2023 Schellenberg, Matits, Bizjak, Kersten, Kirsten, Vollrath and Steinacker. This is an open-access article distributed under the terms of the Creative Commons Attribution License (CC BY). The use, distribution or reproduction in other forums is permitted, provided the original author(s) and the copyright owner(s) are credited and that the original publication in this journal is cited, in accordance with accepted academic practice. No use, distribution or reproduction is permitted which does not comply with these terms.
*Correspondence: J. Schellenberg jana.schellenberg@uniklinik-ulm.de